Avocado Skin Topography
Laurie Meadows
29 August 2019
Some avocado fruit, such as Mexicola, Topa Topa, and Rincon, have
smooth skin. The odd one, such as Lula, has ‘almost’ smooth skin.
Most other have skin with varying degrees of ‘roughness’.
Botanically, the bumps on avocado skin are tubercles, as are the
bumps on the skin of other fruit, such as lychee and gourds. Avocado
tubercles are usually described by terms such as warty, rough,
bumpy, or pebbled (further qualified by words such as low, high,
slightly, somewhat, moderately, highly, very). The diverse terms
used to describe avocado skin surface topography struggle to include
two major components – the number of tubercles per unit area of skin
(tubercle density) and height/width (‘prominence’). Minor components
of skin topography include coalescing of tubercles into ridges;
apparently non-tuberculate sharply defined creases; and disorganized
reticulation.
The question are: what are tubercles? Why do they exist? How do they
arise? This article draws together investigations in other fruit
(mainly) which provide, to a degree, plausible initial explanations.
The article was prompted by this Twitter post by Sonia Rios,
Subtropical Horticulture Farm Advisor, University of California,
showing a ‘sectorial chimera’ in a Hass avocado fruit. A mutation at
a very early stage of fruit development causes a zone or sector of
‘smooth’ skin within the otherwise normal ‘rough’ Hass skin.
https://twitter.com/UCCE_SoniaRios/status/1394170828195962891/photo/1
smooth skin section on the left side of a normal ‘bumpy’ Hass fruit.
As most mutations are ‘loss of function’, blocking or diluting the
‘proper’ operation of a gene, it is fair to suppose this mutated
area of skin is due to the direct or indirect blocking of some
signal to the skin that ordinarily results in the warty tubercles.
Mutations usually happen in the cells of the actively dividing
meristem tissues. The various causes of mutations causing chimeras
and bud sports are extensively listed in Foster
and Aranzana (2018) .
Chimeras in the avocado Skin
According to Foster and Aranzana (2018), angiosperms meristems
consist of (generally) 2 outer layers of cells, the tunica, draped
over an inner layer, the corpus. The first, outer, layer of the
tunica gives rise to the epidermis. The second layer gives rise to
sub-epidermal tissues.
Foster and Aranzana (2018) propose color mutations in one sector of
a flower are due to a mutation in gene expression in a mericlinal
(pole to pole) sectorial chimera affecting either one or both of the
tunicate layers. This is illustrated by the pink petal in the
advanced flower bud of a white azalea cultivar which serendipitously
appeared when I was writing this article. Both the solid color
flower (on the left) and the petal mutant are probable L1 mutants (
Foster and Aranzana, 2018).
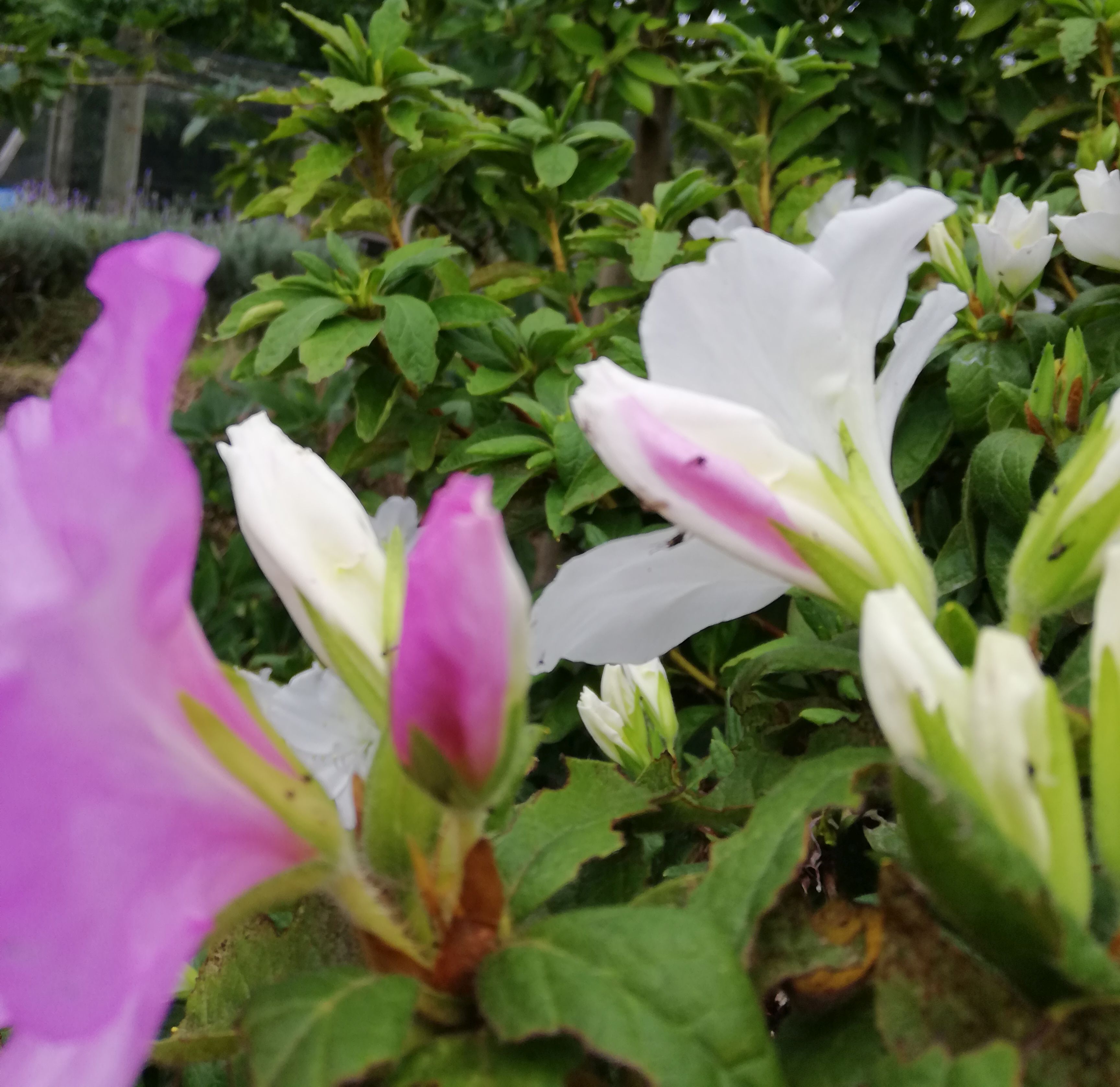
Mericlinal sectorial chimara in a white azalea.
This principle may also apply a gene (or genes) responsible for the
smooth skin sectorial mericlinal chimera in the above photo. This
might imply that ‘pebbled’, ‘bumpy’, ‘warty’ or ‘rough’ skin
topography in Hass and other avocados with various degrees of
tubercule expression is controlled by a single gene, perhaps
additively expressed in the outer tunica.
But, anatomically, what is a tubercle anyway, and how does it arise?
This is a suprisingly complex question to find a plausible answer
to.
Does stomata patterning cause or coincide with tubercle formation?
According to Schroeder (1950), in avocado, the epidermis is made up
of a single layer of cells. A second layer, the hypodermis, either
one or two cells thick, is usually present immediately under the
epidermis. These cells have a natural polymer, cutin, laid down in
the cell walls. Under that are parenchyma cells. Stomata are formed
by a pair of crescent-shaped epidermal cells (the guard cells)
flanking an open pore in the epidermis.
In avocado, stomata have immediately underneath them a mass of
loosely packed cells with abundant intercellular spaces. This is
believed to make gas exchange maximally efficient.
Schroeder notes that in round relatively smooth skinned avocados the
uniform expansion of the skin surface of the growing fruit results
in relatively evenly spaced stomata. But in rough skinned genotypes
the stomata are “restricted to the elevated mounds of
tissues…varieties such as Hass and Dickinson have stomata
concentrated in groups on the elevated surfaces of the rind”.
Curiously, Everett et al (2001) – contra Schroeder – found in ‘firm’
green avocados that lenticels (identifiable “as small yellow dots”)
“form all over the fruit surface, not just on the lumps.” I examined
new season spring-set Hass, Fuerte, 3 seedlings of unknown parentage
and a ‘smooth’ skin cultivar in late autumn and found stomata
(yellow or cream dots) co-incide to the highest degree with elevated
skin relief. If we use a ‘mountain and valley’ analogy, stomata
(presumably aka lenticels) are almost entirely confined to mountain
slopes and are relatively rare on valley floors.
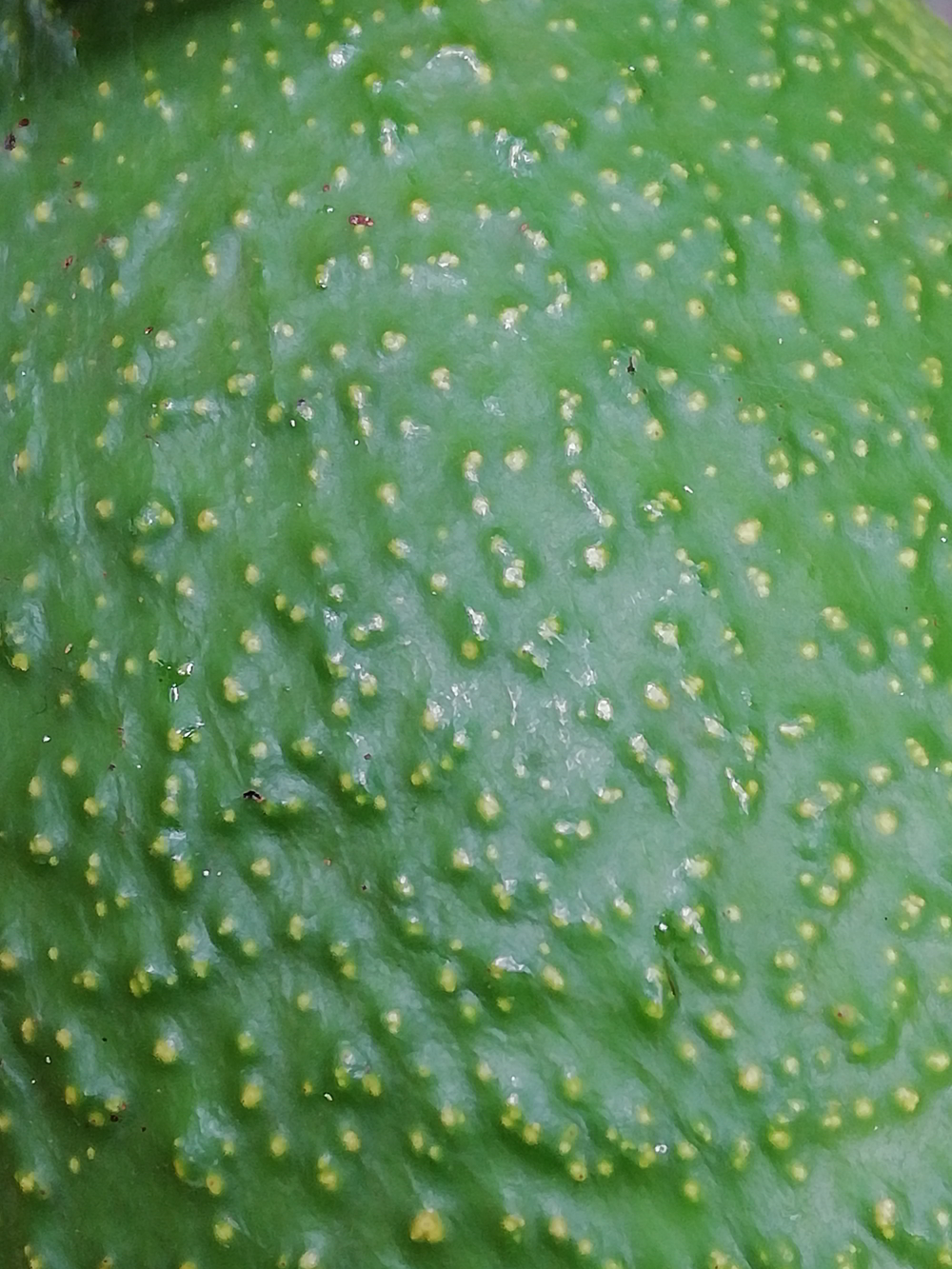
stomata grouped on Hass tubercles
But in immature fruit there is almost no ‘valley floor space’ and
the toe of one ‘mountain’ abuts the toe of adjacent ‘mountains’.
This changes as the fruit increases in size. The valley floors that
’emerge’ are easier to observe. (This is best seen in natural light
where the shadows cast by surface relief across the fruit surface
make topography easy to observe.)
Late-hung last season Hass fruit observed at this stage have lower
relief, that is, ‘flattened’ mountains and wider valley. Creasing,
unrelated to stomata patterning, becomes either more developed, or
more apparent.
The case for tubercle formation being related to tissues associated
with stomata looks promising. So what is the importance of clustered
stomata?
In leaves, Dow et al (2014) define the “proper spacing” of stomata
(on Arabidopsis leaves) as less than 5% of stomata in clusters. This
spacing (scaling with increasing stomata number) achieves maximum
water vapor conductance, maximum gas exchange and consequent net
carbon assimilation, as well as maximal stomatal responsiveness to
increases in CO2 concentration.
In contrast, the authors found that genotypes with greater than 19%
of stomata in clusters had (> 19% clustering) did not. Genotypes
with clustering also had reduced net carbon assimilation and
“impaired” stomatal response to increasing CO2 concentrations, and
impaired stomatal responses. Water use efficiency was largely
unaffected by clustering.
A mutation in a gene or genes controlling the stomatal patterning
process could cause clustering.The clustering phenomenon occurs when
the protodermal cells in the epidermis which produce stomatal guard
cells fail to divide asymmetrically. Asymmetrical division creates
cells between guard cells, spacing out stomata. Symmetrical division
creates two abutting guard cells with no intervening spacing cell.
Clustering also occurs where the pattern of guard cell placement has
an error, and the two adjacent protoderm cells create guard cells on
sections of cell wall immediately facing each other (see
figure 1 Dow et al). This is a simplistic explanation,
and for detail, see Ohashi-Ito
and Bergmann (2006).
If the pattern of stomata placement map to tubercles, then, so long
as the tissues under the stomata are raised or swollen, this may
explain tubercles. Everett et al (2001) found that the
loose gas-exchange tissues under stomata have the ability to swell
with water after heavy rain. The water is not absorbed through the
stomata themselves, it is water absorbed by the roots and pushed by
vascular turgor pressure into the fruit. As the loose sub-stomatal
cells become turgid with water, they act somewhat as a
shock-absorber. About two hours after the rain the cells return to
normal. This temporary cell expansion could hardly explain tubercles
– especially as they are already laid down at the earliest time of
fruit development, regardless of whether it is dry or wet at the
time.
In addition, the number of stomata per square centimeter varies
widely between cultivars. Hass, a genotype with prominent tubercles,
has an average of 69 stomata per square centimeter, and Topa Topa,
which has smooth skin, has about 442 (Schroeder (1950)). This is the
opposite of what you would expect if stomata patterning was
responsible for tubercles.
And as Schroeder also points out, for the most part, stomata on
avocado fruit skin are level with the epidermal layer.
Schroeder showed that a cork cambium and corky tissues develop at
the stylar end of the avocado fruit (commonly called the fruit base,
as opposed to neck) in some genotypes. This is associated with
breakdown and suberisation of the tissues under the stomata. So,
while there can be swelling associated with corky sub-stomatal
tissue, it is only at a late stage of fruit maturity.
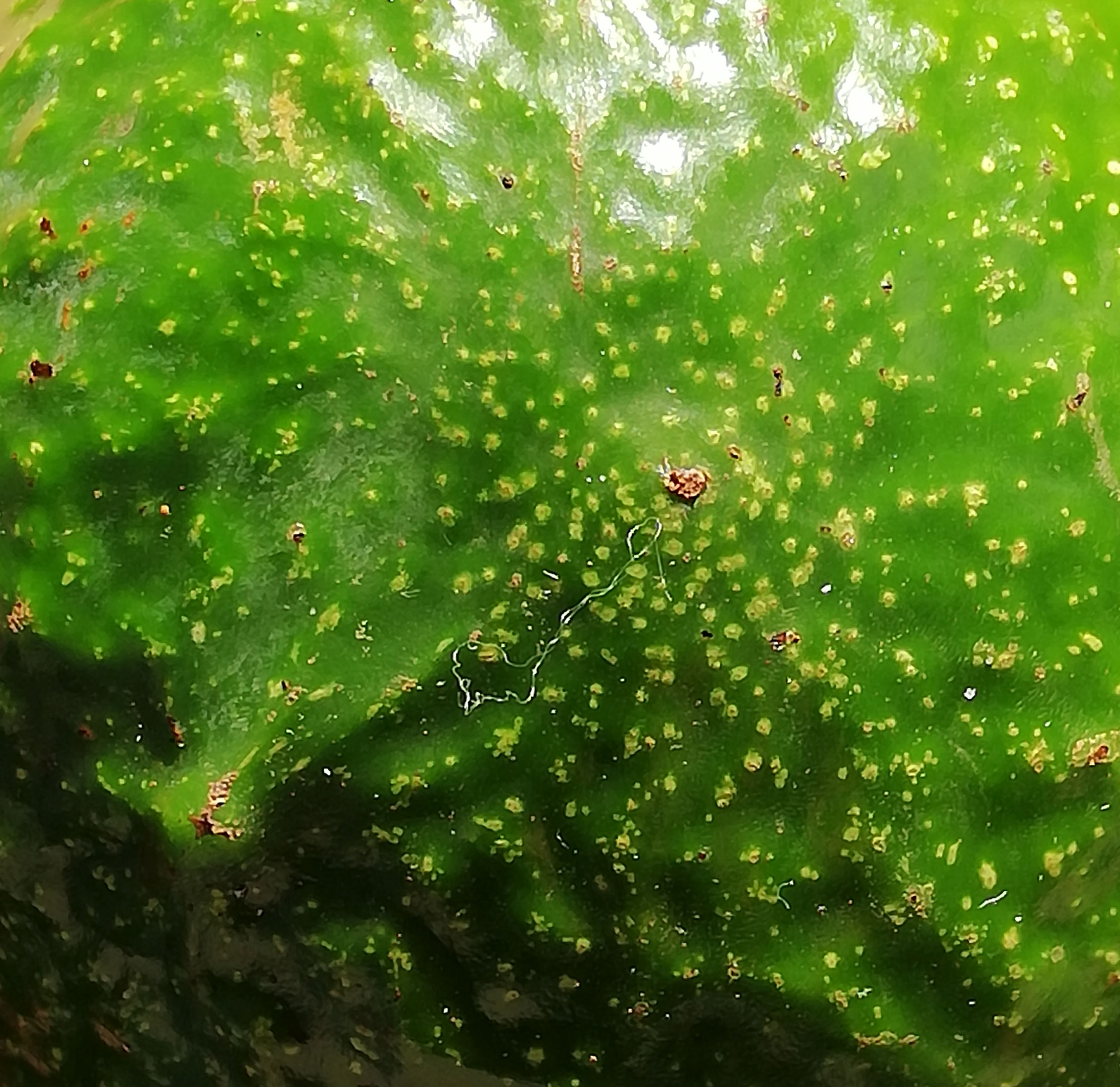
Stomata heavily concentrated around the remnants of the flower style
Finally, stomata are highly concentrated at the stylar end, and in
conditions where these suberise at maturity, they appear to coalesce
into a general corkiness of the base. The corkiness in no way
resembles tubercles, and is localised to the base. In spite of the
high local concentration of stomata, the base is no more
tuberculated than the rest of the skin – and sometimes is less so,
especially in the immediate vicinity of the stylar scar.
That’s it for the ‘stomatal tissue patterning’ hypothesis for
tubercle development – or so it seems.
Rathod et al (2019) investigated the cause of both the
tubercles and the ridges characteristic of the fruit of bitter
gourd, Momordica charantia. They found that both phenomena
were under single gene control. One gene, Tb, controls
tubercles, and another, Cr, controls whether ridges are
discontinuous, rather than continuous.
In cucumber, Cucumis sativa, a functionally identical gene
(isofunctional homologue) also causing tubercles is labelled Tu
(CsTu).
The Tb gene.
Crosses of smooth-skinned and ‘warty’ (tuberculate) genotypes of
bitter gourd showed tuberculate to be dominant over smooth (Kole et
al 2012). Similarly, the discontinuous ridge character state (Cr)
was found to be dominant over continuous ridges (cr).
A Tb – like gene in avocado
An avocado isofunctional homolog of this gene would explain the
surface topography of avocado fruit. It is also permissive of some
association with the gene/s associated with stomata patterning (if
so, this would be pleasantly tidy).
The variation in expression of tubercle height in avocado plants
might be the result of the Tb gene being additive. A cross
between a tuberculate cultivar and a cultivar that has a smooth skin
as a result of a mutation that prevents Tb expression (tb
mutant) fits nicely with this scenario, and Sonia Rios’s
presentation of a smooth sector chimera in a tuberculate avocado
fruit provides convincing evidence.
Selfed Hass seedlings show both markedly tuberculate progeny, and
progeny with nearly smooth skin (Bergh and Whitsell 1973). If Tb
was dominant in avocado, all the progeny would have had markedly
tuberculate skin.
This result would be explained if Hass was heterozygous for Tb
(Tb/tb). Absent modification by other genes, Tb/tb
x Tb/tb ‘should’ give one Tb homozygote with
markedly rough skinned seedling and one tb homozygote with
apparently smooth skin to every two heterozygotes with intermediate
skin pebbling.
In contrast to Hass, Fuerte has relatively small and low tubercles.
But Bergh and Whitsell (1974) record that “many” selfed Fuerte
seedling have “warty” skin, which suggests Fuerte, too, is a
heterozygote for this character.
But why does Hass express the Tb gene so strongly if it is a
heterozygote with only one active copy of the gene?
There may be other genes that further modify Tb expression.
How the Tb gene might work in avocado
Yang et al (2014) discovered that in cucumber, the Tu gene codes for
a transcription factor (a C2H2 zinc finger protein) that ‘probably’
promotes cytokinin biosynthesis in the tissue that ultimately became
tubercles. This transcription factor is further enhanced by another
gene, Tsl, which affects the ultimate size of tubercles.
Tubercle size – the Ts1 gene
The specific gene (Ts1) listed in Wang et al 2020
(supplementary file 1) that specifies cucumber tubercle size codes
for an oleosin protein, and is a promoter of gene expression. There
are low levels of Ts1 expression in cucumbers without warts,
or with small warts, and high levels of expression in those
cultivars and lines with greater tubercle size (Yang et al
2019).
This heritable variation in tubercle size due to expression of the Tsl
gene has a strong appeal as a plausible cause of variability in
tubercle size in heterozygote avocado cultivars.
Some avocado cultivars, and Maluma is a good example, are extremely
tuberculate. This is exaggerated at the immature stage.
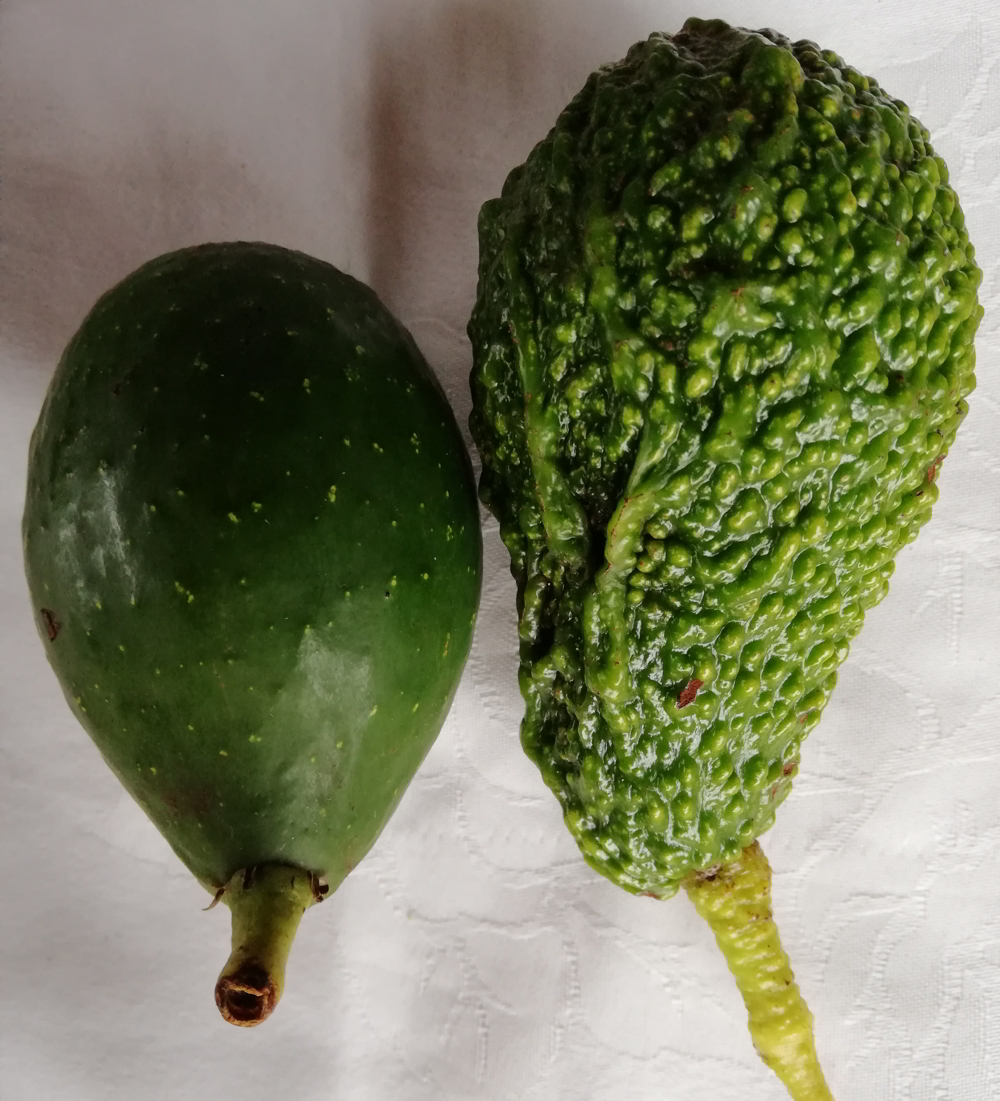
Left, seedling showing low tubercle expression, right Maluma
immature fruit with ‘hyper-expression’
This kind of ‘hyper-expression’ may well be due to even further
epigenetic influences that enhance tubercle size.
Within-fruit localised tubercle over-expression
Avocado fruit – or at least pyriform avocado fruit – are
asymmetrical. If you stand an avocado up on its base it may look
symmetrical, but in fact the morphological ‘true’ base point of the
fruit, at the floral scar (stylar scar, strictly speaking), is
shifted up the side of the fruit, away from the apparent base
(Cummings and Schroeder, 1942; see Fig. 12). The stylar scar, the
‘true’ halfway point, is marked with a yellow pin in the photo of
the late season Hass fruit below. In other words, one side of the
fruit is ‘smeared around the bottom of the fruit, visually at the
expense of the other half.
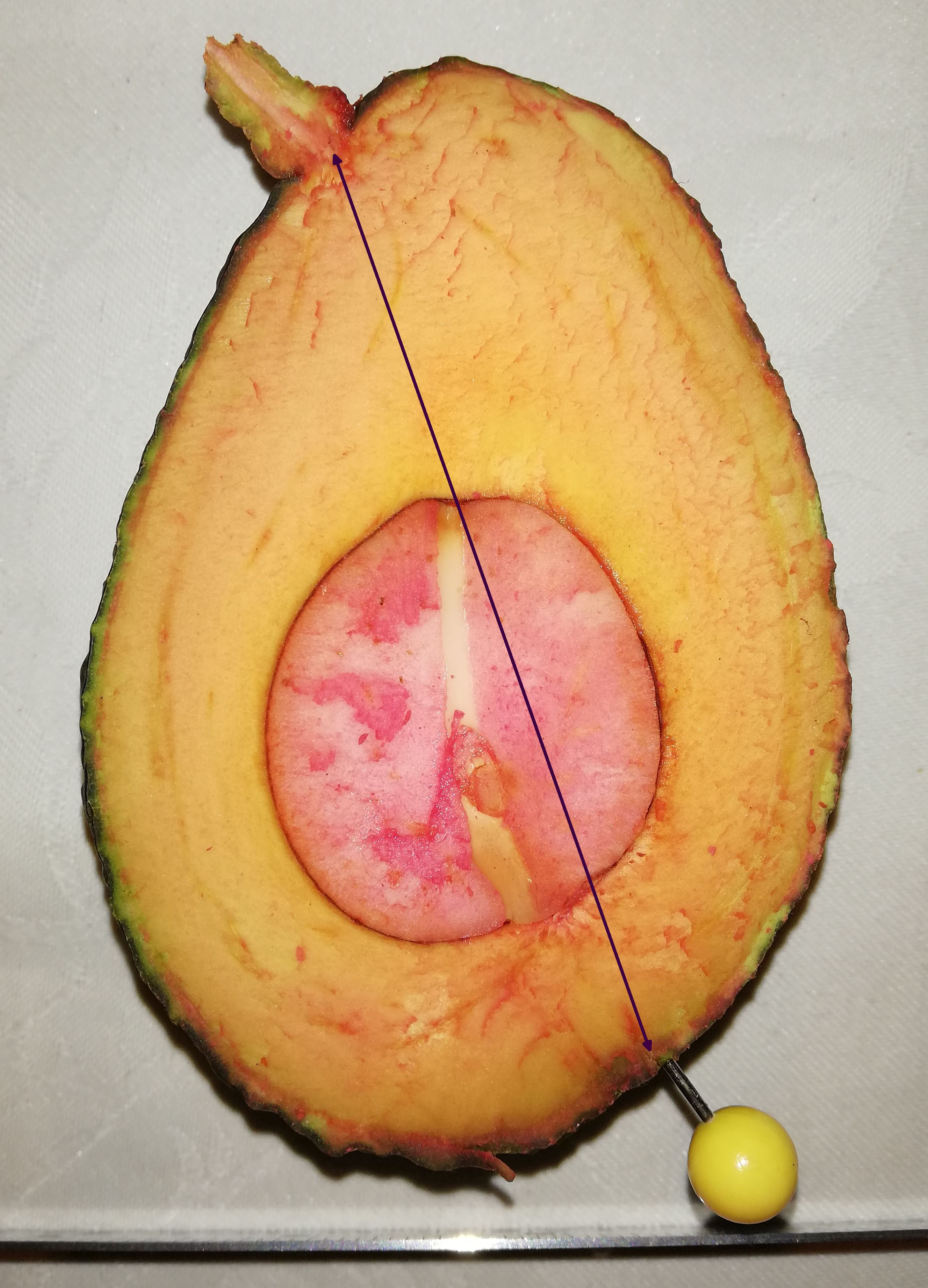
The ‘apparent’ short (left hand) side of this Hass is has about the
same perimeter distance as the other side.
The fruit stalk is attached offset from the apex in many pyriform
cultivars, and viewed from the bottom of the stalk to the apparent
base, this creates an apparent ‘short side’. The apparent short side
meridial sector has significantly greater tubercle expression than
the rest of the fruit surface, especially the apparent ‘long’ side.
The ‘long’ side has rather flattened tubercles, which becomes more
marked as the fruit enlarges and matures.
But the perimeter of the botanically accurate sides, measured from
the pedicel core to the stylar scar, are almost identical in the
above illustrated mature Hass fruit. The more heavily tuberculated
‘short side has a perimeter of 124 mm, and the ‘long’ side is 127
mm. Of course this is only one measurement and one cultivar.
For clues as to why side of the fruit might be more heavily
tuberculated, we can look to the internal morphology of the fruit,
which is well described in Cummings and Schroeder, 1942. In short,
one half, the apparent short side has more and better vasculature,
and is in contact with the seed as it develops. The apparent short
side is more important to the plant for this very reason – it feeds
the seed, it is the pipeline to the tree.
In contrast, the apparent ‘long’ side had fewer ‘pipes’, thinner
‘pipes’, and its vasculature terminates at the floral scar (or ends
blindly), and doesn’t enter the seed at all (Cummings and Schroeder,
1942).
It is plausible that both endogenous hormones from the tree and
locally produced phytohormones are delivered more quickly and more
efficiently to the apparent short side, increasing signal
concentration, and ‘pumping up’ tubercles locally. In the meantime,
the infrastructurally depauperate apparent long side is
short-changed in phytohormone supply and has reduced tubercle
expression as a result.
This leaves still open the questions of which tissues initiate
tubercle formation, and what exactly is a tubercle anyway.
What are tubercles?
Yang et al (2014) showed that in cucumber, tubercle
initiation starts two days before flowering and continues until
thirteen days after flowering. Tubercles develop due to localised
rapid cell division. But it isn’t epidermal cells that develop into
tubercles, spine cells do. Tubercles develop directly below the
layer of spine cells. See Yang et al‘s (2014) paper for
details.
Site of gene expression
Yang et al‘s (2014) work confirmed that in the crucial
tubercle initiation stage 2 days before flowering, the cucumber Tu
gene was not expressed in the epidermal tissues, nor in the tubercle
tissues, but only in the basal cell layers of the spine. However, Ts1
which interacts with the Tu gene is expressed in tubercles,
epidermis and pulp adjacent to the epidermis (Yang et al 2019).
This implies signalling by a phytohormone. Cytokinin, involved in
cell proliferation and differentiation is a good candidate.
The authors observed that the smooth skinned cucumber lines had
small, fine spines (really a specialised form of trichome), whereas
the ‘warty’ lines had large robust spines.
But avocado fruit don’t appear to have spines, remnant or not, as
far as my x20 eyeglass allows me to see (although Wang et al
2020 mention what they call a “micro-trichome”(mict)
mutant that shows no visible trichomes on fruit). Schroeder (1950),
who examined the skin morphology in detail, makes no mention of
hairs, spines or structures atop of tubercles. Everett et al describes
lenticels, but makes no mention of spines.
Avocado don’t seem to have spines, and are therefore absent the
layer of spine basal tissue that proliferates to form tubercles. But
they do have areas of tissue proliferation on the skin. These areas
of initially undifferentiated cells are the meristemoid tissues that
are triggered to go on to form stomata in a patterned manner.
A speculative mechanism of tubercle origin in avocado
Mutations in the stomata precursor cells cause poorly controlled
cell division, forming, in some cases ‘epidermal ‘tumors’ of guard
cells (see Fig.2 (C) of Ohashi-Ito and Bergmann (2006) for an
example in Arabidopsis leaf). The pattern, or map of the way
stomata ‘should’ appear is also disturbed.
It is plausible that in avocado a similar series of mutations in
cell proliferation and mapping might result in a clustered pattern
of stomata whose underlaying tissues and adjacent cells have
receptors sensitive to cytokinins (and maybe auxins). The cytokinins
then cause proliferation of cells, and therefore tubercles. At some
point the complex hierarchy of gene feedback then down-regulates
promoters, cytokinin levels fall away, and tubercle growth stops.
What selective advantage do tuberculate fruit have over non
tuberculate fruit?
There might be biomechanical advantages if tubercles ‘flatten’ with
swelling fruit. Sudden increases in turgor after heavy rain can
cause fruit skin cracking. A combination of cutin deposits in the
epidermis (perhaps for tensile strength), sclerenchyma for skin
thickness, and tubercles for flexibility might prevent premature
cracking and fruit drop. The seed can then reach full maturity with
maximal reserves in the cotyledons.
But avocado seeds are usually viable a long before full natural tree
ripeness, so this seems a weak argument.
So far, I see no satisfactory explanation.
References
Bergh B. O., and Whitsell R. H. 1973. Self -Pollinated Hass
Seedlings.
California Avocado Society 1973 Yearbook, 57: 118 -156
Bergh B. O., and Whitsell R. H. 1974. Self -Pollinated Fuerte
Seedlings.
California Avocado Society 1974 Yearbook, 58: 128-134
Cummings, K, and and Schroeder C. A. 1942. Anatomy of the Avocado
Fruit.
California Avocado Society 1942 Yearbook 27: 56-64
Dow, G.J., Berry, J.A. and Bergmann, D.C. (2014), The physiological
importance of developmental mechanisms that enforce proper stomatal
spacing in Arabidopsis thaliana.
New Phytol, 201: 1205-1217. https://doi.org/10.1111/nph.12586
Everett, K. R., Hallett, I. C., Yearsley, N., Lallu, N.,
Rees-George, J., Pak, H. 2001. Lenticel Damage.
NZ Avocado Growers Association Annual Research Report Vol. 1 2001
Foster, T. and Aranzana, M. 2018. Attention sports fans! The
far-reaching contributions of bud sport mutants to horticulture and
plant biology
Horticulture Research 2018 5:44
Doi 10.1038/s41438-018-0062-x
Huang, X. M., Yuan, W. Q., Wang, H. C., Li, J. G., Huang, H. B.,
Shi, L., & Jinhua, Y. (2004). Linking cracking resistance and
fruit desiccation rate to pericarp structure in litchi (Litchi
chinensis Sonn.).
Journal of Horticultural Science and Biotechnology, 79, 897-905.
doi: 10.1080/14620316.2004.11511863
Kole C., Bode A. O., Kole1 P., Ra V. K., Bajpai A., Backiyarani S.,
Singh J., Elanchezhian R. and Abbott A. G. 2012. The first genetic
map and positions of major fruit trait loci of bitter melon (Momordica
charantia). J. Plant Sci. Mol. Breed., 1(1): 1-6. Doi:
10.7243/2050-2389-1-1.
Ohashi-Ito, K., and Bergmann, D. C. (2006). Arabidopsis FAMA
controls the final proliferation/differentiation switch during
stomatal development. Plant Cell 18, 2493–2505. doi:
10.1105/tpc.106.046136
Rathod, V., Behera, T. K., Gaikwad, A. B., and Hussain, Z. 2019.
Genetic analysis and tagging of gene controlling fruit tubercles and
fruit ridgeness pattern in bitter gourd using SSR markers.
Indian J. Genet., 79(4) 749-755 (2019) DOI: 10.31742/IJGPB.79.4.14
Schroeder, C. A. 1950. The Structure of the Skin or Rind of the
avocado.
California Avocado Society 1950 Yearbook 34: 169-176
Wang, Y., Bo, K., Gu, X. et al. Molecularly tagged genes and
quantitative trait loci in cucumber with recommendations for QTL
nomenclature. Hortic Res 7, 3 (2020). https://doi.org/10.1038/s41438-019-0226-3
Yang, S., Wen, C., Liu, B., Cai, Y., Xue, S., Bartholomew, E.S.,
Dong, M., Jian, C., Xu, S., Wang, T., Qi, W., Pang, J., Ma, D., Liu,
X. and Ren, H. 2019. A CsTu–TS1 regulatory module promotes
fruit tubercule formation in cucumber.
Plant Biotechnol J, 17: 289-301. https://doi.org/10.1111/pbi.12977
Yang X, Zhang W., He H., Nie J., Bie B., Zhao J., Ren G., Li Y.,
Zhang D., Pan J., and Cai, R. 2014. Tuberculate fruit gene Tu
encodes a C2H2 zinc finger protein that is required for the warty
fruit phenotype in cucumber (Cucumis sativus L.).
Plant J. 2014 Jun;78(6):1034-46. doi: 10.1111/tpj.12531